The Secret Science Behind Graphene’s Gas Sensing Capabilities
- thefxigroup
- Feb 27
- 4 min read
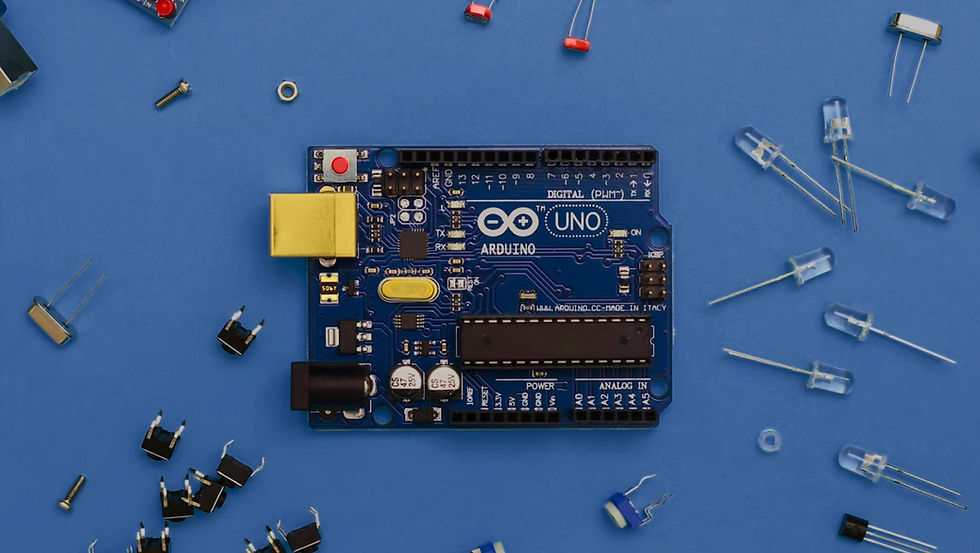
Graphene has garnered significant attention for its amazing electrical, mechanical and chemical properties and has many potential applications. In recent times, graphene is being sort out for use as a gas sensor due to its exceptional sensitivity, rapid response time and ability to operate at room temperature. Graphene’s unique characteristics make it a prime choice for detecting a wide range of gases, from toxic industrial pollutants to biomarkers in medical diagnostics.
One of the main reasons graphene excels as a gas sensor is its high surface-area-to-volume ratio. Unlike bulk materials, where only a fraction of the atoms is exposed to the environment, every atom in a graphene sheet is available for interaction with gas molecules, maximizing the potential for absorption and enhancing sensitivity. Since graphene is a zero-bandgap semiconductor with high electron mobility, even the adsorption of a single molecule can alter its electrical conductivity in a detectable way. What this means is that graphene-based sensors can operate with an extremely low detection limit, making them suitable for applications where even trace amounts of gas must be identified.
Another advantage of graphene gas sensors is their ability to function without requiring high operating temperatures. Traditional metal oxide gas sensors, for example, rely on elevated temperatures, typically above 200°C, to achieve sufficient sensitivity. This high-temperature requirement increases power consumption and limits application in portable or wearable devices. In contrast, graphene-based sensors can detect gases at room temperature, making them highly energy-efficient and suitable for integration into compact and low-power electronic systems. This property is particularly valuable for applications in environmental monitoring, industrial safety and medical diagnostics, where continuous, low-power operation is necessary.
The mechanism behind gas detection in graphene sensors is mostly based on charge transfer and electrostatic interactions between gas molecules and graphene surface. Different gases act as electron donors or acceptors, causing measurable changes in graphene’s electrical resistance or conductance. For instance, electron-withdrawing gases such as nitrogen dioxide increase resistance while electron-donating gases such as ammonia decrease it. This change in resistance can be precisely measured allowing the sensor to determine both the presence and concentration of specific gases. The inherent stability of graphene also ensures that these interactions are reversible, enabling repeated used of the sensor without significant degradation.
Despite these advantages, pristine graphene does have some limitations as a gas sensor, due to its relatively weak gas adsorption properties and lack of sensitivity. In its unmodified form, graphene tends to interact with various gas molecules in a similar manner, making it challenging to distinguish between the different gases. To address this issue, researchers have explored a variety of strategies to enhance graphene’s selectivity and sensitivity. A common approach is the functionalization of graphene with nanoparticles, metal oxides and organic molecules. By attaching specific chemical groups or nanostructures to the graphene surface, researchers can create selective binding sites that preferentially interact with target gases. For example, decorating graphene with palladium nanoparticles enhances its ability to detect hydrogen while functionalization with metal oxides like tin dioxide improves sensitivity to carbon monoxide.
Another method to improve graphene’s gas sensing performance is doping, where foreign atoms like nitrogen, boron, or fluorine are introduced into the graphene lattice. Doping alters graphene’s electronic properties and can enhance its interaction with certain gas molecules, thereby improving selectivity and detection efficiency. Similarly, the creation of defects or introducing strain in the graphene sheet can modify its adsorption characteristics, further optimizing its sensing capabilities.
In addition to chemical modifications, device engineering also plays a crucial role in optimizing graphene-based gas sensors. Various configurations, such as field-effect transistors and resistive sensors, have been developed to maximize graphene’s response to gas exposure. Graphene FET-based sensors, for instance, offer highly sensitive real-time detection by leveraging the gate voltage to modulate sensor response. This approach enables precise control over sensor performance, allowing for tailored sensitivity to specific gases. Moreover, integrating graphene sensors with flexible substrates and wireless communication technologies has opened new possibilities for wearable and IoT-enabled gas sensing applications.
Graphene’s rapid response and recovery times further enhance its appeal as a gas sensor. Unlike conventional materials that may take minutes to react to gas exposure, graphene-based sensors can detect changes within seconds or even milliseconds. This ultrafast response makes them suitable for applications where real-time monitoring is essential, such as detecting gas leaks in industrial settings or monitoring air quality in urban environments. Furthermore, their quick recovery time allows for continuous and repeated use, increasing their practicality in long-term sensing applications.
The potential applications of graphene gas sensors are vast and span multiple industries. In environmental monitoring, they can be used to detect air pollutants such as nitrogen oxides, carbon monoxide, and volatile organic compounds, contributing to improved air quality management. In the healthcare sector, graphene-based sensors hold promise for non-invasive disease diagnostics by detecting biomarkers in breath analysis. For example, certain volatile organic compounds present in human breath have been linked to diseases such as lung cancer and diabetes, and graphene sensors could enable early detection through breath analysis. Moreover, in industrial safety, these sensors can provide real-time alerts for hazardous gas leaks, preventing workplace accidents and improving worker safety.
With ongoing advancements in functionalization, device engineering, and large-scale production, graphene-based gas sensors are poised to revolutionize multiple industries, from environmental monitoring to healthcare and industrial safety. As research progresses, these sensors will likely play an increasingly important role in shaping the future of gas detection technologies.